Michael W. Crowder
Miami University. Department of Chemistry
crowdemw@miamioh.edu
sites.google.com/a/miamioh.edu/crowderlab
One important area of research in our lab is the development of techniques to probe mechanism of inhibitor for new MBL inhibitors. We can readily determine if an MBL inhibitor forms a ternary complex or if it strips metal from an MBL. Our lab is open to collaboration with other groups in order to investigate the mechanism of inhibition of potential inhibitors. Recently synthesized inhibitors can be shipped to our lab for characterization using the following techniques: inductively coupled plasma with atomic emission spectroscopy (ICP-AES); nuclear magnetic resonance spectroscopy; UV-Visible spectroscopy; electron paramagnetic resonance spectroscopy and native-state electrospray ionization mass spectrometry. Below are explanations of each technique, the information gained, and an example of potential results for the characterization of the mechanism of inhibition.
Equilibrium dialysis and metal analyses:
In this experiment, we incubate the MBL with varying concentrations of inhibitor, and then we dialyze the enzyme/inhibitor mixture versus buffer. The resulting sample is then analyzed for metal content by using either inductively coupled plasma with atomic emission spectroscopy (ICP-AES) or atomic absorption spectroscopy. Interpretation of the data are straightforward; the metal content of the mixture versus the concentration of the added inhibitor yield plots as shown below. Inhibitors (such as EDTA) that have a metal-stripping mechanism of inhibition will show a reduction in metal content, while those inhibitors (such as captopril) that have a metal coordination mechanism will show no loss of metal. Limitations of this technique are that large amounts of enzyme and inhibitor are needed. For example, our ICP-AES is configured to need enzyme concentrations of 10 µM or greater; therefore, a single experiment with five different inhibitor concentrations will require 6 mg of MBL and 10 mg of inhibitor. Another limitation is that the inhibitor must be water-soluble up to 100-200 µM and stable in non-HEPES buffers.
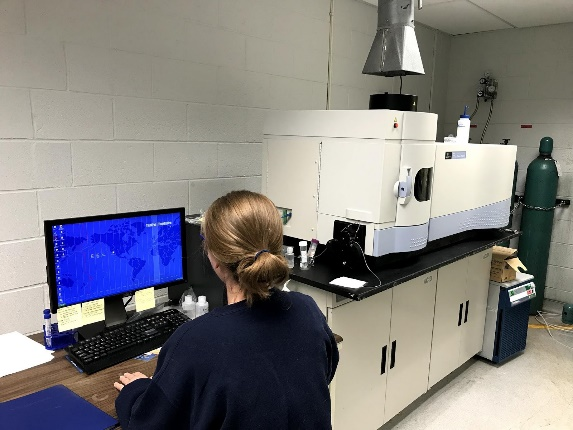
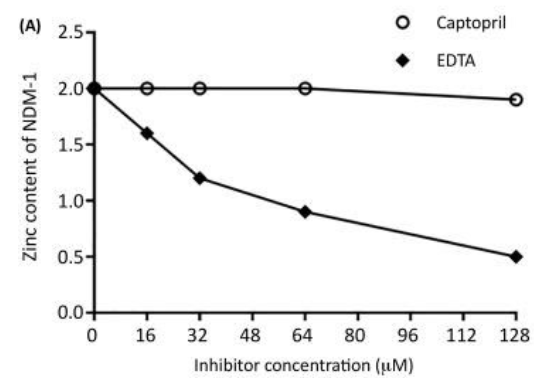
ESI-Mass Spectrometry (native-state MS):
An important challenge in interpreting equilibrium dialysis/metal analyses experiments is the relatively large concentrations of enzyme/inhibitor necessary to conduct the experiments. Cellular concentrations of an effective inhibitor will most likely be in the low nanomolar range, while the concentrations of inhibitor in these experiments are in the micromolar range. An inhibitor that is a metal stripping agent at high concentrations could possibly form ternary complexes at lower concentrations, depending on the relative Kd values for metal binding of the inhibitor as compared to that of the enzyme. We are currently developing a native-state mass spectrometric method to analyze the equilibrium dialysis samples (see below).
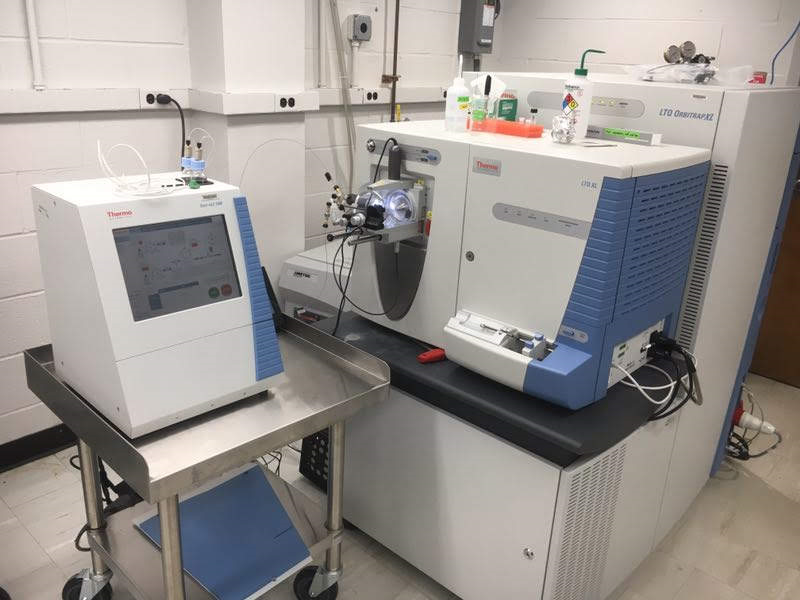
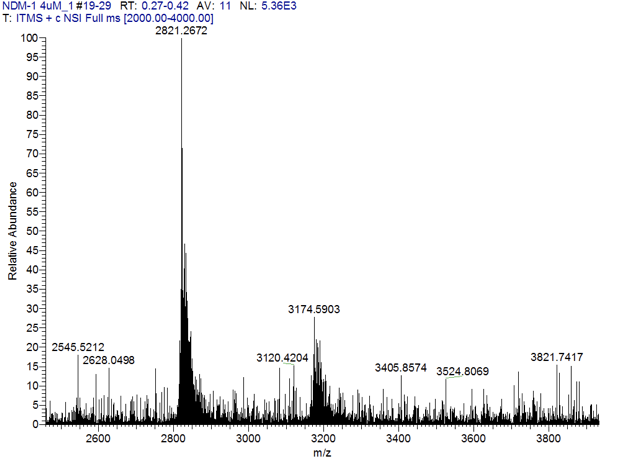
NMR Spectroscopy:
Building on over 2 decades of spectroscopy on MBLs1-7, we are developing techniques to probe MBL/inhibitor ternary complexes. All of the common techniques require large quantities of Co(II)-substituted MBLs, which have been shown to be excellent surrogates of the naturally-occurring MBLs. 1H NMR spectroscopy has been used to identify which residues are bound to Co(II) in MBLs and thus can be used to probe any changes of the metal binding ligands upon MBL inhibitor binding8-9. If an inhibitor forms a ternary complex, the resulting spectra will often show shifted peaks; whereas, if an inhibitor strips the MBL of Co(II), the spectra will lack shifted 1H resonances and present new peaks that represent the Co(II)-inhibitor complex. This latter information has been used to confirm equilibrium dialysis experiments and mechanism of inhibition. One important note on NMR spectroscopy is that it requires millimolar enzyme and inhibitor concentrations. It is very possible that an inhibitor that utilizes a metal stripping mechanism of inhibition at millimolar concentrations could utilize a ternary complex forming mechanism at micromolar concentrations.
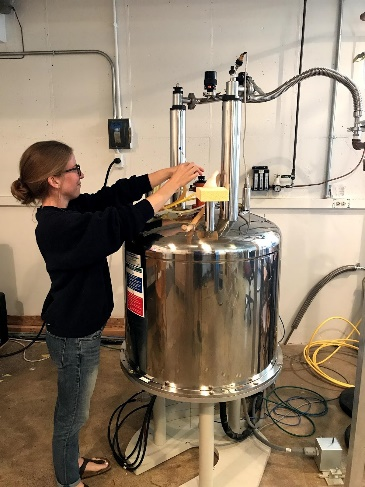
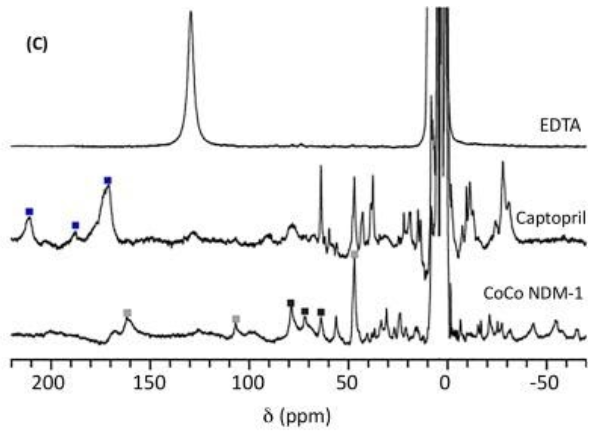
Above is an example of the data collected on a 300 MHz 1H NMR spectrometer of CoCo-NDM-1 with two equivalents of EDTA (top), CoCo-NDM-1 with three equivalents of captopril (middle), and CoCo-NDM-1 (bottom). Because there are two metal ions within the active site, peaks were labeled to demonstrate which peaks are associated with metal binding ligands in the Zn1 site (black squares) or in the Zn2 site (gray squares). The peaks marked with blue squares indicate protons associated with residues in a ternary complex between NDM-1-Co(II) and captopril. Any loss of peaks in the NMR spectrum indicate the inhibitor removed Co(II) from the active site (shown with EDTA).
UV-Vis Spectrophotometry
Similar to our NMR studies, UV-Vis studies with MBLs must utilize Co(II)-substituted analogs of the enzymes. UV-Vis spectrophotometry can provide two important pieces of information about inhibitor binding to Co(II) in the MBLs. First, most Co(II)-substituted MBLs exhibit an intense UV-Vis peak at 320-350 nm due to a cysteine sulfur ligand to Co(II) metal charge transfer (LMCT) transition4. If an inhibitor causes the Co(II) to dissociate from the active site, this peak will disappear. In addition, if binding of the inhibitor causes changes in the orientation of the cysteine-Co(II) bond, these changes will be reflected in changes in the intensity and/or wavelength of the LMCT transition. Second, there is a series of 3-4 peaks from 500-650 nm, which are assigned to the d-d or ligand field transitions of high-spin Co(II) 4. Previous studies have shown that the intensities of these peaks is dependent on the coordination number of the Co(II) ions, with 4-coordinate Co(II) being the most intense, 6-coordinate Co(II) being the least intense, and 5-coordinate Co(II) being in the middle4. If the inhibitor binds to the Co(II) in this site and causes a change in the coordination number of Co(II), the d-d peaks could be altered.
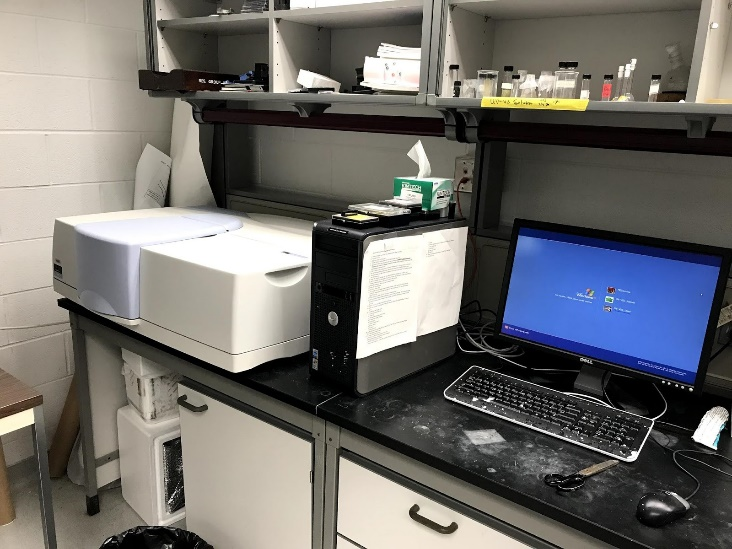
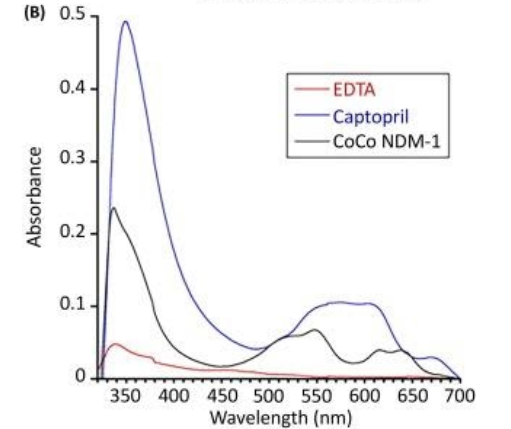
EPR Spectroscopy:
Another technique that uses Co(II)-MBL metalloforms to characterize ternary MBL/inhibitor complexes is electron paramagnetic resonance (EPR) spectroscopy. To obtain EPR signals on high-spin Co(II) containing samples, the spectra must be obtained at low temperatures (<30 K) and at protein concentrations between 0.2 and 1 mM. Typically, EPR signals for Co(II)-substituted MBL are broad with small changes in the signal on binding of an inhibitor to the metal ions. But, if the inhibitor bridges the Co(II) ions, the spin coupling between the Co(II) ions can change and will be detected with EPR spectroscopy when run in both parallel and perpendicular modes10
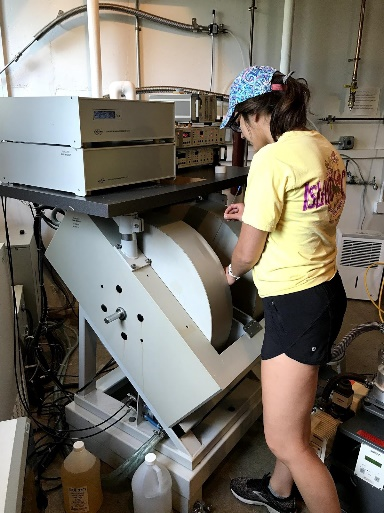
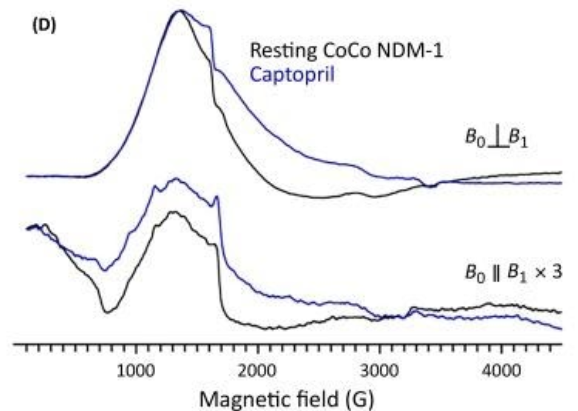
References
1. Lisa, M. N.; Palacios, A. R.; Aitha, M.; Gonzalez, M. M.; Moreno, D. M.; Crowder, M. W.; Bonomo, R. A.; Spencer, J.; Tierney, D. L.; Llarrull, L. I.; Vila, A. J., A general reaction mechanism for carbapenem hydrolysis by mononuclear and binuclear metallo-beta-lactamases. Nat Commun 2017, 8 (1), 538.
2. Aitha, M.; Al-Adbul-Wahid, S.; Tierney, D. L.; Crowder, M. W., Probing substrate binding to the metal binding sites in metallo-beta-lactamase L1 during catalysis. Medchemcomm 2016, 7 (1), 194-201.
3. Aitha, M.; Marts, A. R.; Bergstrom, A.; Moller, A. J.; Moritz, L.; Turner, L.; Nix, J. C.; Bonomo, R. A.; Page, R. C.; Tierney, D. L.; Crowder, M. W., Biochemical, mechanistic, and spectroscopic characterization of metallo-beta-lactamase VIM-2. Biochemistry 2014, 53 (46), 7321-31.
4. Yang, H.; Aitha, M.; Marts, A. R.; Hetrick, A.; Bennett, B.; Crowder, M. W.; Tierney, D. L., Spectroscopic and mechanistic studies of heterodimetallic forms of metallo-beta-lactamase NDM-1. J Am Chem Soc 2014, 136 (20), 7273-85.
5. Griffin, D. H.; Richmond, T. K.; Sanchez, C.; Moller, A. J.; Breece, R. M.; Tierney, D. L.; Bennett, B.; Crowder, M. W., Structural and kinetic studies on metallo-beta-lactamase IMP-1. Biochemistry 2011, 50 (42), 9125-34.
6. Sharma, N.; Hu, Z.; Crowder, M. W.; Bennett, B., Conformational changes in the metallo-beta-lactamase ImiS during the catalytic reaction: an EPR spectrokinetic study of Co(II)-spin label interactions. J Am Chem Soc 2008, 130 (26), 8215-22.
7. Periyannan, G. R.; Costello, A. L.; Tierney, D. L.; Yang, K. W.; Bennett, B.; Crowder, M. W., Sequential binding of cobalt(II) to metallo-beta-lactamase CcrA. Biochemistry 2006, 45 (4), 1313-20.
8. Hu, Z.; Periyannan, G.; Bennett, B.; Crowder, M. W., Role of the Zn1 and Zn2 sites in metallo-beta-lactamase L1. J Am Chem Soc 2008, 130 (43), 14207-16.
9. Bertini, I.; Luchinat, C.; Parigi, G.; Pierattelli, R., Perspectives in paramagnetic NMR of metalloproteins. Dalton Trans 2008, (29), 3782-90.
10. Chen, A. Y.; Thomas, P. W.; Stewart, A. C.; Bergstrom, A.; Cheng, Z.; Miller, C.; Bethel, C. R.; Marshall, S. H.; Credille, C. V.; Riley, C. L.; Page, R. C.; Bonomo, R. A.; Crowder, M. W.; Tierney, D. L.; Fast, W.; Cohen, S. M., Dipicolinic Acid Derivatives as Inhibitors of New Delhi Metallo-beta-lactamase-1. J Med Chem 2017, 60 (17), 7267-7283.